10,000 RPM Spindle Upgrade - Overview
On my previous upgrade to the G0704, I designed a moderately complicated spindle belt drive and pneumatic drawbar assembly. This took advantage of a 1.8kW, 5000 RPM, 4.2 Nm continuous torque, 11.1 Nm peak torque, 90mm frame AC servo I had access to. I chose to use GT3 5mm pitch curvilinear timing belts for synchronous motion (needed for rigid tapping) and smooth running even at high speed. With a later addition of some serial scripting to the spindle drive, I added a real time load monitor for the spindle. I have been absolutely thrilled by this modification and remains one of the most successful designs I've built.
For years I've been using this and contemplating the most optimal spindle design for a mill of this size. I'll link to a couple discussions we had here on this site.
Measuring tool offsets offline with BT30 is tricky too. Haven't tried it yet, but I'm not so sure about that. I think I'll turn a chunk of steel to have a BT30 ID taper and face both ends. This goes on the surface plate with the socket taper facing up. Stick a tool holder in the spindle and...
www.hobby-machinist.com
Hi All, I have owned a CNC converted G0704 for about 9 years now and really have a ton of hours on it. One of the things I really enjoy thinking about and have done some experimenting with is understanding how to overcome the significant limitations of these smaller machines by selecting...
www.hobby-machinist.com
My uses of this machine seem to revolve around 90% aluminum and plastics, and probably 10% steel and cast iron. My most used tool is a 3/8" YG-1 3 flute aluminum cutting endmill. I have found that in 95% of cases I am programming the mill spindle to 5000 RPM at all times. Watching the spindle load meter during my machining, I rarely see it above 20%. This leads me to the conclusion that I did a poor job of selecting the belt ratio for the machine. Fortunately, my belt drive design left it easy to modify and replace the pulleys as needed to achieve various speed configurations.
Doing some math, I decided to try to optimize the spindle for use of the YG-1 3/8" endmill and smaller tools. This sacrifices the low end torque of the machine, but again I rarely need that anyways and I should be able to replace the use of large diameter tools with higher performance mid-sized tools. Here is the speeds and feeds chart for the endmill I use the most.
We see the recommended side profiling speeds and feeds call for 8000rpm and ~100 ipm @ 25% radial engagement and 100% axial engagement. I've found that my machine runs best at around 15% radial engagement and 200-300% axial engagement with this tool. My normal speeds and feeds are 5000 rpm and 40 ipm.
Using the speeds and feeds calculator, we can see what horsepower and torque are needed for the cut. I've previously measured my total spindle efficiency of 92% and use a unit power for 6061-T6 aluminum and a honed edge tool of 0.4 HP/in^3/min.
2.45 HP equates to exactly 1.8 kW (the continuous rating of my servo), and 17.7 in-lb is equal to 2.0 Nm of torque. With a 1:2 belt drive, this cut would be in the continuous power regime of the motor (meaning I have the full 1.8kW available) and the motor should be able to produce 2.1 Nm torque continuously. Honestly I think the machine rigidity and coolant requirements would limit this cut before the spindle load does.
This leads me to the conclusion that the 1:2 belt drive configuration is ideal for 3/8" and smaller tooling in aluminum. The spindle should have plenty of torque for milling steel, where I am significantly more limited in machine rigidity than spindle power. I will suffer in the torque needed for larger drills. Some rough calculations show that where I could push a 1/2" drill before, I will now be limited to roughly a 3/8" drill under the same cutting conditions. I rarely use drills over 1/4" and when I do need to, I can adjust the cutting parameters to account for the torque limitations.
In the same line of thought, lets consider rigid tapping (not that I've even actually used it). My 1:1 spindle could offer 4.2 Nm (37 in-lb) continuous torque, and the proposed 2:1 spindle offers 2.1 Nm (18.6 in-lb). The table below shows common tapping torques for sharp ground taps:
So, hypothetically I should be able to still drive a 1/4" tap in brass, 3/8" in aluminum, and #10 in mild steel. The spindle will happily offer 300% torque for a short while if needed. The 1:2 spindle maintains the ability to orient and index the spindle via the spindle motor marker, allowing for pack tapping if needed. other ratios such as 2:3 would not permit this.
OK, so cool, I'm going with a 1:2 spindle belt ratio. I like to purchase pulleys from SDP-SI and they have a wide variety of offerings for the GT3 5mm size. The main limitation is the small pulley needs to fit over the spindle spline shaft and have keys installed. This creates a minimum size pulley for the driven side of 22T. This means the larger pulley will need to be 44T. I did not plan ahead very well and did not leave sufficient clearance in the spindle housing for the pulley. It will need to be removed and opened up a bit for better clearance. Since this will take the mill out of commission, I needed a friend to give me a hand with the machining.
Before:
After:
The large driver pulley is not offered with flanges, however a lower flange is required for vertical installation such as this. I will need to design, machine, and install a lower flange so the belt cannot fall off the bottom of the pulley.
Before I go further, I wanted to check that the belt can sufficiently handle the loads and speeds of the application. Gates (the PowerGrip GT3 manufacturer and licenser) has a great design manual
HERE.
The speed/power chart shows that the GT3 5mm pitch belt is suitable for 10,000 rpm at the faster pulley and up to 10HP. Additional notes in the manual suggest the top speed to be around 14,000 rpm with special design considerations.
In the Flange Design section of the manual, the following guidance is offered for vertical installation of belts:
"On vertical shaft drives, one sprocket should be flanged on both sides, and all the other sprockets in the system should be flanged on the bottom side only."
Finally we need to make sure that the minimum teeth in engagement and belt wrap angle are respected. For this the following guidance is offered:
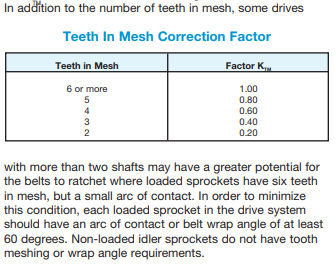
Using their online calculator, I was able to select the required pulleys and belts. Notice that both pulleys have >6 teeth in mesh and a wrap angle >60 degrees. The belt length (75T) was selected to give as close to 4.05" center to center distance. This means the new belt drive will fit into the spindle housing with no further modifications needed beyond opening the housing for a bit more clearance.
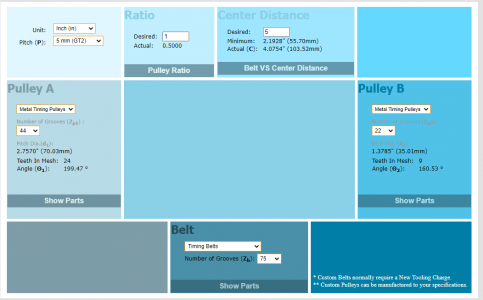
With these changes, I've left myself room to install up to a 50T driver pulley allowing up to 12,000 RPM (with a small tweak on the servo speed maximum on the drive). However, I don't think that any additional speed will be worth the torque tradeoff.
Overall the pulleys should be fairly straightforward to machine, and I'll post updates here.
There are a few concerns with the higher spindle speeds:
Thermal heating in the bearings and belts creating higher than desired temperature rise in headstock. The spindle currently stabilizes at ~110*F @ 5000 rpm. This will increase substantially with higher speed.
Spindle balance. The R8 spindle is not balanced and additional speed will increase vibrations. I will need to take care to keep everything concentric to my best ability.
Bearing wear will be increased. The angular contact and deep groove bearings selected have a maximum speed of 14,000 RPM with grease, but the higher speed will increase wear.
TTS tool holders are limited to 10,000 RPM according to Tormach.
I've sent a boring head to 5000 RPM before on accident and it was terrifying. 10,000 RPM could make that situation downright dangerous.
Reduced spindle clamp force. At higher speeds, the centrifugal force on the petals of the R8 collet will tend to make them "open" and decrease the clamping force on the tool shank. I am investigating increasing the drawbar clamp force.
Noise will be significantly increased. The downside of the synchronous timing belt is that it runs much louder than say a poly-V belt. The GT3 curvilinear tooth profile and nylon fabric face on the belt do quite a lot to reduce running noise, but I expect 10,000 RPM will get quite loud. I will maintain the airtight belt drive housing to help with this and may consider adding felt facing to the interior walls of the belt housing for additional sound damping.
That's all for the moment.
-Mike